Archived Coronavirus Pandemic Questions
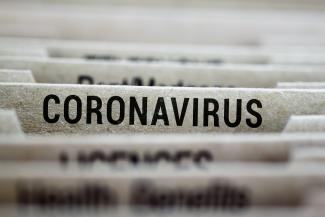
On this page, you will find an archive of the questions and answers addressed during 2020 and 2021 on “The Coronavirus Pandemic – Answering Your Questions” page. To note: Archived answers to questions were up to date at the time of publication but are not continuously updated. The archive serves solely as a record of past questions and answers.
We also have a page dedicated to coronavirus questions on the VEC website. Go to COVIDVaccineAnswers.org for even more information.
May 12, 2021
What is a vaccine mandate?
See if you can identify which of these statements are true:
- It is illegal to drive faster than the posted speed limit.
- Certain home building projects require construction permits.
- If a restaurant does not pass a health inspection, the inspectors can close it.
Did you guess that all of these are true? If so, you are right! Each of these reflect rules and laws designed to keep people safe from things like car accidents, unstable structures, and foodborne illnesses. Another name for a rule or law is a mandate.
Mandates in everyday life
While mandating something may sound like an uncommon occurrence, mandates are part of our everyday lives. Take the example of traffic laws. Speed limits are mandates designed to make drivers, passengers, and the people around them safer. While in certain rare circumstances it may be acceptable to significantly exceed the speed limit (e.g., an ambulance speeding to the hospital during a medical emergency), it is generally unacceptable and illegal to drive faster than the limit. Speed limits set a standard to prevent people from endangering themselves or others. Similarly, drivers and passengers are mandated to wear seatbelts. In the event of an accident, people are less likely to get severely injured or die if they are wearing a seatbelt. In this way, seatbelts improve the safety and health of everyone. Vaccine mandates are similar; they require that certain groups of people receive a vaccine to keep themselves from getting a disease and to prevent them from spreading it to others. As such, vaccine mandates also keep everyone safer and healthier.
Mandates are actually quite common for vaccinations, even if they aren’t always called by that name. Many schools and colleges require students to receive certain vaccines before they are allowed to attend classes. These requirements are designed to reduce the chance of infectious diseases spreading in classrooms. Not only does this keep students healthier, but it also protects their families because students are less likely to bring an infectious disease home. Similarly, many hospitals have employee mandates for the yearly influenza vaccine to not only keep their employees safe, but also their patients.
Immunization mandates may also be implemented during outbreaks or pandemics because they can help stop the spread of a disease in a community or population. Typically, to effectively stop the spread of a disease, the population needs to reach “herd immunity.” Herd immunity, also called “community immunity,” is the percentage of the population that needs to be immune to a contagious infection to stop its ability to effectively spread from one person to another. Individuals can only become immune by having the illness or getting vaccinated. Since isolating all people with the disease involves far more resources than vaccination, vaccination is the preferred way to achieve herd immunity. However, getting most of the population vaccinated, particularly in a short amount of time, requires many individuals to work together to achieve the common goal. Unfortunately, some people within the group may not be able to be vaccinated. Others may simply choose not to be vaccinated. If enough people opt out of vaccination, herd immunity will not be achieved. If herd immunity is not reached, the disease will continue to spread, increasing the risk for everyone. As such, vaccine mandates may be needed to reach the necessary percentage to achieve community immunity.
While the science behind vaccine mandates is clear, they also provide an example of the complexity of science and society. Because some individuals may not want to get a vaccine, making a law requiring it may be viewed as limiting an individual’s freedom of choice—even though they are making this choice for others with whom they come in contact. Nonetheless, some people may determine that they are unlikely to get the disease, so they don’t want the vaccine. Or, they may be concerned about the vaccine’s safety, so they feel the risk of the vaccine is greater than the risk of the disease. They may have religious beliefs that the vaccine conflicts with, such as if a particular ingredient is included. Or, they may simply want the right to choose whether to get vaccinated. For these individuals, mandates will be more about their emotional and personal views than the science involved in protecting the public.
Vaccine passports
In the same way that vaccine mandates may become emotionally charged, so, too, may something called “vaccine passports.” You may have heard of these during the COVID-19 pandemic. So, what are they and how do they relate to vaccine mandates?
Vaccine passport is a term that refers to documentation or proof of vaccination. Such documentation would permit the holder to participate in activities that nonvaccinated individuals might not be allowed to. Proof of vaccination is currently required for entry into countries where yellow fever is common.
While deciding when and how to use COVID-19 vaccine passports may be complicated in a manner like implementing vaccine mandates, the logic behind these passports is straightforward. People who are immunized are much less likely to get or spread COVID-19 to other people. Because of this, a gathering of vaccinated people is likely to be safer than a gathering of unvaccinated people. As such, as businesses and event venues work to reopen, they will likely want to do so in a way that decreases the chance for people to get exposed to COVID-19. By asking visitors to show a vaccine passport, businesses can hold safer gatherings and help people understand the level of risk posed by attending an activity or event. The use of vaccine passports would likely vary in different situations. However, vaccine passports could be used as a tool to allow people to get back to activities and events more quickly and safely. They could be used in the same way proof of a negative coronavirus test has been required in certain situations during the COVID-19 pandemic.
While the term “vaccine passport” may be unfamiliar, many examples exist in our society where people must have documentation to legally participate in activities. For example, a driver’s license is required to operate an automobile. A passport is required to prove an individual’s identity and nationality when entering a foreign country. A birth certificate is required for school enrollment or to procure a marriage license. And proof of vaccination is required for school entry. Despite these existing uses, vaccine passports have caused concerns during the COVID-19 pandemic. It is not yet clear whether or how widely they will be implemented.
Summary
Like other laws that protect people, vaccine mandates are designed to prevent the spread of infectious diseases in a population. Sometimes mandates are necessary to reach herd immunity if enough people do not voluntarily get vaccinated. Whether mandates will be needed for COVID-19 remains to be seen; however, as the country and the world tries to cautiously return to normalcy, vaccine passports and mandates could be tools that help us get through the pandemic.
Think about it:
- List two other examples of laws designed to keep people safe, and if you think the laws accomplish that goal.
- Pick a community stakeholder and describe two or three reasons why they might support vaccine mandates or passports.
- The article lists examples of why some people object to vaccine mandates. List one or two other examples of objections you think people may have to vaccine mandates or vaccine passports.
- Do you think an individual should have the freedom of choice to comply even if that choice could cause harm to another person? Why or why not?
Related resources
Human Immune System: Types of Immunity, VEC
Unit 2 Lesson 2: Discovery and Development of Vaccines, VMP
This lesson focuses on the different ways that people become immune to pathogens
and includes activities and resources related to herd immunity.
April 21, 2021
How do we know the COVID-19 vaccines work?
In the United States, there is a clear and comprehensive system of clinical trials in place. Clinical trials are studies that test a vaccine in phases, starting with small groups of healthy adults and eventually expanding to larger groups of many different types of people. This system helps ensure that a vaccine is safe and works before it can be approved and recommended for widespread use.
We talked about how we know vaccines are safe in the December 22, 2020 entry, “How do we know COVID-19 vaccines are safe?” In this entry, we will focus on how we know vaccines work by discussing two important terms, “vaccine efficacy” and “vaccine effectiveness.”
Vaccine efficacy
The official term for how well a vaccine or treatment works in a clinical trial is known as “efficacy.” To test a vaccine, researchers conduct double-blind, placebo-controlled clinical trials. So, what does this mean?
- Double-blind means that the study is set up such that neither the participants nor the researchers know who got the vaccine. This is important because it decreases the chance that people’s biases affect the results. For example, if a person knows they got a medicine, they might feel better than if they did not get it. Feeling better might be because the medicine worked, but it could also be because they know they had medicine. This is called a “placebo effect” because studies have shown that even people who get a placebo treatment sometimes feel better.
Since people in clinical trials need to report how they feel, double-blinding controls for the placebo effect. Likewise, the people running the study do not know who got the vaccine and who didn't, so that they do not inadvertently treat people in the two groups differently or interpret the side effects or experiences of participants differently.
- Placebo-controlled means that study volunteers are randomly split into two groups. One group, called the experimental group, receives an injection of the vaccine being tested. The other group, called the control group, receives a placebo. The placebo is a benign substance that looks like and can be administered in the same way as the vaccine. In COVID-19 clinical trials the placebo was saline, which is a salt solution. You can see examples of other types of placebos in the “Phase III trials” part of this webpage.
After the volunteers receive the vaccine or the placebo, they are monitored over time. Often, the participants are asked to keep notes about any health-related matters. That information helps researchers determine which side effects occur and how often, and they watch for any vaccine safety concerns. Researchers also monitor how many people get the disease during the trial. Scientists “break the code” to see who was in the vaccine group and who was in the placebo group after a pre-determined time interval or after a certain number of people become sick.
Below are two sample sets of vaccine trial data. In each case, 100 people got the vaccine and 100 people got placebo. In both examples, 30 of 100 people (or 30%) who received the placebo got the disease. We can use the data to tell us if the vaccine was effective.
Example 1 – Vaccine A
In this example, 30 people who got vaccine A got sick and 70 remained healthy. This means 30% of the people who received vaccine got sick. When we compare this to the placebo, it is the same — 30% of the placebo group got sick. The lack of difference between the Vaccine A and placebo groups indicates that Vaccine A did not work. At this point, development of Vaccine A would likely stop because it did not offer any protection from disease.
Example 2 – Vaccine B
In this example, 5 people who got Vaccine B became sick and 95 remained healthy, so 5% of the people who received the vaccine got sick. In the placebo group, 30% of the people got sick. The lower percentage of illness suggests that Vaccine B protected people against the disease over the time period of the study.
Using information like that shown in examples A and B, scientists can calculate vaccine efficacy. Vaccine efficacy is the percent reduction of disease in the clinical trial population following vaccination. To calculate this number, scientists use this equation:
Historically, a few vaccines have had the opposite of their intended effect and made people more susceptible to disease. The vaccine trials were stopped as soon as this was realized. This is why clinical trials are so important. They are designed to discover issues with a vaccine before it is approved for use by the public. For vaccines, clinical trials are big enough to detect major issues, but sometimes an issue will not become clear until millions of people have been vaccinated.
Vaccine effectiveness
In the above examples, we looked at vaccine efficacy, or how well the vaccine worked in the context of a clinical trial. However, a vaccine may not work as well when it is used in the real world. This is known as “vaccine effectiveness.”
Watch a short video about the difference between vaccine efficacy and vaccine effectiveness.
Reasons for differences between efficacy and effectiveness can include:
- Differences between people who volunteered in the study and the general population, particularly because often in clinical trials people must meet certain criteria to participate.
- Differences between conditions in the study and those in the real world. For example, the study population may have been limited to a certain age group that on average is younger than the age range of those receiving the vaccine in the real world. Since the immune system weakens over time, when older people use the vaccine, they may not be as well protected.
- Greater variation in the dosing schedule in the real world. For example, in a clinical trial, participants are scheduled for their appointments according to the study protocol, but in the real world, people’s schedules vary greatly. People may miss appointments or not be available at the exact follow-up time, or the office may not have an appointment at the recommended time. The net result is that the timing during which doses of vaccine are given will likely be more variable.
- Similarly, during vaccine trials only clinical sites that are approved to participate in the trial are giving the vaccine. Most often, this means a limited number of staff members are involved, and, therefore, vaccine storage, handling, and administration is fairly standardized. But, once a vaccine is being administered throughout the country, the number of locations and healthcare personnel handling the vaccine will be greater, and, therefore, more variability in storage, handling, and administration will naturally occur.
Over time, scientists examine the data from real world conditions to determine a vaccine’s effectiveness and compare it to the efficacy. This helps them to better understand the vaccine and make sure it is working similarly to what would be expected based on the clinical trials.
Summary
The clinical trial process allows scientists to evaluate whether a vaccine is safe and works before it is distributed to the public at large. Clinical trials also give us a measurement to describe how well the vaccine works, called its efficacy. But, it is not until a vaccine is administered to the general population that we get a better understanding of how effective the vaccine is in real world conditions, called vaccine effectiveness. Both of these measures are important for scientists and public health officials working to keep people healthy.
Think about it:
- Would you volunteer for a vaccine clinical trial? Why or why not?
- If your job was to recruit volunteers for a vaccine clinical trial, what would be important for participants to know?
- Can you think of other factors that might influence vaccine effectiveness in the real world?
- Practice calculating vaccine efficacies. Worksheet | Answers
- Do you think different vaccine efficacies might be acceptable based on circumstances? Explain your answer.
Related resources
Making Vaccines: Process of Vaccine Development, VEC
How do we know COVID-19 vaccines are safe?, VMP
What Is the Difference between Vaccine Efficacy and Vaccine Effectiveness? (Video), VEC
March 18, 2021
What can teens expect when getting a COVID-19 vaccine?
(Updated 6/15/2021)
Are you excited to get a COVID-19 vaccine? Maybe a bit nervous? People have different feelings about getting vaccines — and individuals even sometimes have different feelings about different vaccines. An array of feelings is normal, but sometimes it helps knowing what to expect.
Teen vaccine eligibility
As of May 2021, three COVID-19 vaccines are authorized for use in the United States — Pfizer-BioNTech, Moderna, and Johnson & Johnson/Janssen. Of these, only the Pfizer vaccine is approved for ages 12 and older, with the other two vaccines currently approved for ages 18 and older. Clinical trials determined that the Pfizer vaccine is safe and works well in those 12 years and older. Clinical trials of the Moderna and Johnson & Johnson vaccines are underway for teens and children, and Pfizer is currently testing their vaccine in those younger than 12 years of age.
Preparing to get the vaccine
Most immunization sites require appointments though some may have “walk-in” hours for eligible people to get vaccinated. This varies widely from state to state, so you should check how things are being done in your area if you are now eligible to be vaccinated.
A few other considerations are important before going for a COVID-19 vaccine:
- If you have a history of severe allergic reactions (i.e., you carry an “epi pen”), a compromised immune system, or a health condition that might interfere with getting the vaccine, check with your healthcare provider to figure out if you can get the COVID-19 vaccine. You may be able to get it, but need to take special precautions. For example, some people are recommended to wait for 30 minutes of observation instead of 15 minutes after getting the vaccine.
- Unless you regularly take a pain-relieving medication prescribed by your doctor, it is not recommended to take a pain reliever (such as ibuprofen or acetaminophen) before vaccination. These types of medications may affect how well the vaccine works. However, it is important not to stop taking a medication prescribed by your doctor before getting the vaccine without first checking that it would be safe to do so.
- You may go to a vaccination site in your community, a local pharmacy, or your doctor’s office to get the vaccine. This also depends on how your state is handling COVID-19 vaccine distribution.
What to expect when getting the vaccine
If you are going to get a COVID-19 vaccine, here is an idea of what you may experience:
- Before you go to the immunization site, remember to wear a mask and plan to social distance the same way you would in any other public space during the pandemic. Wear clothing that makes it easy to access your upper arm without removing your shirt. Take any appointment confirmation, identification, or other items requested by the site. You may also want to take a book or your phone to pass time spent waiting. In some cases, you may be asked to read information about the vaccine and complete a form on the computer before you go to the appointment.
- When you arrive, you will most likely be asked to sign in or confirm your appointment and eligibility. The health care professionals at the site will probably ask you and your parent or guardian a few questions before you get the vaccine. For example, they may ask if you have any history of severe allergic reaction or if you are allergic to certain things.
- After you are signed in, you may need to wait for your turn to get vaccinated. Waiting areas should be set up with social distancing measures to ensure everyone’s safety.
- When it is your turn, the person giving the vaccine may ask you a few more questions. For example, they might confirm your name, ask in which arm you want the vaccine, or repeat questions about allergies or other vaccines. This repetition helps ensure that people are safely and appropriately vaccinated. It is particularly important at vaccination clinics where many people are coming and going.
- When the vaccine is administered, it does not take long and usually feels like a pinch. Many people find it helpful to distract themselves during the shot by looking at something across the room or talking to someone. If you feel nervous about getting the vaccine, you could also try listening to music or playing with an app to distract yourself. Other ways to decrease your nervousness and the “pinch” include taking a few slow, deep breaths as the vaccine is given, or ask for an alcohol pad to be rubbed on your opposite wrist shortly before the vaccine is administered. As the vaccine is given, blow on the area with the alcohol; you will feel the cool temperature of the alcohol evaporating more than the pinch of the shot.
- After the shot is complete, you will most likely be asked to go to a waiting area. While the risk of an allergic reaction from a COVID-19 vaccine is very low, it makes sense for everyone to be observed after the injection as a safety precaution. People are asked to wait 15 to 30 minutes in case they have any allergic reactions to the vaccine. Although rare, these reactions can occur. They typically occur soon after getting the vaccine, which is why people are asked to wait.
- At some point during the appointment, you should receive a vaccination card that tells you which COVID-19 vaccine you received and the date you received it. Some people immediately take a picture of this card, so if it is misplaced, they still have the information. While it may be tempting to post a picture of your vaccination card on social media, it is not recommended to do so as the card may have personal information that should not be made public. Some vaccination sites have selfie stations or offer stickers that provide alternative ways to share your vaccination experience with your followers.
- Since the Pfizer vaccine is a two-dose vaccine, you may also be asked to sign up for a second appointment. The site will let you know the correct timing for your second injection. Many sites do the second appointment scheduling during the post-vaccine observation period.
- You may also be asked if you are up to date on other vaccines, so that you can get those while you are getting the COVID-19 vaccine. But it is not clear if all vaccination sites will be offering this service. Teens may need vaccines such as Tdap, HPV, or meningococcal vaccines (one or both versions). Some may also need to catch up on vaccines typically given at younger ages, like MMR, hepatitis A, hepatitis B, polio, or chickenpox. While getting multiple vaccines may make you nervous, particularly if you don’t like shots, don’t let the possibility deter you from getting your COVID-19 vaccine. Remember, getting a vaccine is much faster and less painful than any of these diseases.
What to expect after getting the vaccine
Getting a COVID-19 vaccine is similar to other immunizations. Some people have side effects while others do not. Side effects are a sign that your body is responding to the vaccine, but don’t worry if you don’t have side effects — it doesn’t mean the vaccine didn’t work. People’s immune systems respond differently. The most common side effects are pain, redness or swelling at the injection site or tiredness, low-grade fever, or muscle aches for a day or two after getting the vaccine. For the mRNA vaccines, these side effects tend to be more common after the second dose. A very small number of young people, particularly males, have also experienced a condition called myocarditis. Myocarditis, or inflammation of the heart, is a condition that sometimes occurs after viral infections, including COVID-19. Current data indicate that the condition occurs within 4 days of dose 2 and affects about 1 in 50,000 people between 16-39 years of age. Symptoms may include chest pain, shortness of breath, or a fast heartbeat. If you experience these, or any other symptoms you are unsure of, tell a nearby adult or contact your health care provider. While this condition may sound scary – and be scary if you experience it – it is important to know that it typically goes away in a few days and does not cause permanent heart damage. The CDC is continuing to monitor the situation, but given the risks of the vaccine and the risks of the disease, which more often causes myocarditis, both the CDC and the American Heart Association recommend that eligible teens get the COVID-19 vaccine.
To help scientists monitor vaccine side effects, your parent can sign up for V-Safe to report any side effects that you feel. V-safe is a vaccine monitoring program developed by the CDC. This program will send check-in text messages after each dose of vaccine to gather data on any side effects you may experience. This system helps with continuing to monitor vaccine safety, particularly in different groups of people. Individuals across the country have participated in the program. Hopefully, you will participate, too, so that scientists can gather information about the vaccine experience of young people.
Immune responses develop a couple of weeks after getting the last dose. Right now, we know that most people who completed the full course of coronavirus vaccine are unlikely to get sick from COVID-19. However, we are still studying how long protection lasts. So far, we know it lasts for at least 6 months. Vaccinated individuals should continue to follow the guidance related to mask wearing and social distancing suggested by the CDC and local health authorities.
It may be different for people who are fully vaccinated, which occurs two weeks after getting the second dose. As more people get vaccinated, more restrictions will be lifted. For this reason, it is important that everyone who can get vaccinated do so — not only for themselves, but also for those around them and for the community at large.
Think about it:
- Why do you think it is important to do vaccine clinical trials on teens and children rather than relying on the findings in adults?
- How do you think the experience of a teen receiving a vaccine would be different from that of a parent or other adult?
Related resources
Your COVID-19 Vaccine Appointment, CDC
Age Groups and Vaccines: Teens/College, VEC
Questions and Answers about COVID-19 vaccines, (includes more about myocarditis, including a short video),VEC
Myocarditis and Pericarditis Following mRNA COVID-19 Vaccination, CDC
Download a version of this article updated on 6.15.2021 [PDF, 164 KB]
March 10, 2021
Will the COVID-19 vaccines work on the newer variants of coronavirus?
Scientists hope the answer to the above question will be yes. So far, the limited data we have suggests that the current mRNA vaccines and the newer adenovirus-vector vaccine will be effective against most existing variants. Let’s review how viruses evolve and how vaccines work to understand why these variants are causing concern.
How viruses evolve
Viruses that reproduce in people infiltrate human cells so they can use the cells’ machinery to replicate. Every time a virus reproduces in a cell, its genetic material is copied. During the copying process there is an opportunity for the virus to change or evolve. This can happen in one of two ways:
- Mutation: A virus can change if a mistake occurs when the viral DNA or RNA is copied. For example, information can be left out or entered in the wrong place. RNA viruses tend to be “sloppy” and make mistakes during the copying process that result in mutations.
- Recombination: A virus can change by sharing genetic material with another virus that has infected the cell at the same time.
When a virus changes, the new version of the virus is called a variant. When the variant replicates, that mutation is also replicated. Sometimes, these new variant viruses have an advantage over previous versions. For example, the mutation might help the virus spread from one person to another more easily. When this happens, the variant with the advantageous mutation will be more likely to survive than older versions. In the case of SARS-CoV-2, the virus that causes COVID-19, recent variants have been in the news. Scientists are working to understand if these new versions of the virus are stopped by antibodies produced through vaccination.
Watch this short animation about how coronaviruses evolve.
How a vaccine could stop working against a new variant
First, let’s review how the current COVID-19 vaccines work. These vaccines train the body to recognize and neutralize the spike protein on the surface of the SARS-CoV-2 virus. As part of this immune system training, the body develops antibodies to the spike protein. These antibodies float around in the blood checking to find a protein that they can bind to. Wherever a protein match is found, the antibodies bind to them and prevent the virus from attaching to cells.
Antibodies generated after vaccination might not be able to bind to the spike protein if the virus evolves in a way that changes the shape of the spike. In this manner, the variant virus will no longer be prevented from attaching to the person’s cells. So, how can scientists tell if a new variant can evade existing antibodies?
Scientists can evaluate the ability of the vaccines to stop new variants in a few ways:
- Antibody studies – Scientists can combine antibodies from vaccinated people with new versions of the virus in the lab. They test to see if the antibodies can “neutralize” the virus or stop it from infecting cells. If the antibodies work in the lab, it is likely that they would also stop the virus from infecting vaccinated people in the community. It is important to recognize that while these studies are the fastest to do, they do not tell us everything we need to know. Antibodies are only one part of the body’s immune system defenses. Even if they do not completely neutralize the virus, other immune system components may still be able to protect a vaccinated person from getting sick.
- “Real world” studies – Over time, scientists can monitor people who have been vaccinated or naturally infected. They check to see if they are becoming sick with the different variants despite the immunity that was generated by natural infection or vaccination. These studies are more challenging and expensive to do.
What happens if the vaccines don’t work against the variants?
If scientists find that vaccinated people are not protected against newer variants, they can revise existing vaccines to better match the variants. This is what is done each year for influenza. The influenza virus changes so rapidly that scientists need to make a new version each year. Hopefully, one day scientists will be able to create a so-called “universal” influenza vaccine — one that will protect against influenza no matter how much the virus changes.
As with influenza, scientists are monitoring SARS-CoV-2 and the new COVID-19 vaccines. They are working to create revised vaccines in case the current ones become less effective. If this happens, people might need to get a booster dose. Or, if the virus continues to spread rapidly and change, people may need to get multiple booster or annual doses. Right now, scientists do not think people will need annual COVID-19 vaccines, but time will tell.
Summary
When viruses replicate, they often change. If those changes are advantageous, new versions, called variants, will become more common. As variants arise, they may also be able to evade immunity caused by previous infection or vaccination. In the case of SARS-CoV-2, current research suggests that the COVID-19 vaccines will continue to offer protection, but scientists will continue to monitor variants in case something changes.
Think about it:
- Based on the discussion above, how does the number of people who become infected affect the chance of a new variant forming? How do the number of variants affect the chance of needing to revise vaccines?
- If you were a public health professional, what would be your recommendation(s) for how society could avoid or decrease the opportunity for variants to develop?
- If you were a scientist working on vaccine development, how would you prepare for the chance that variants might arise?
- If a variant formed that our current vaccines didn’t work against at all, what steps do you think would need to be taken to protect people from it?
Related resources
Biology of SARS-CoV-2: Evolution (Animation), HHMI BioInteractive
Emerging SARS-CoV-2 Variants, CDC
Unit 2 Lesson 2: Case Studies: Influenza and HIV, VMP
This lesson focuses on the mechanisms by which viruses infect cells and replicate. The lesson also covers genetic processes by which viruses are able to circumvent immune system defenses.
February 16, 2021
What is the difference in the immune response between vaccinated and unvaccinated people?
Please take a few minutes to review this image and see what you can conclude from it before reading the rest of the passage.
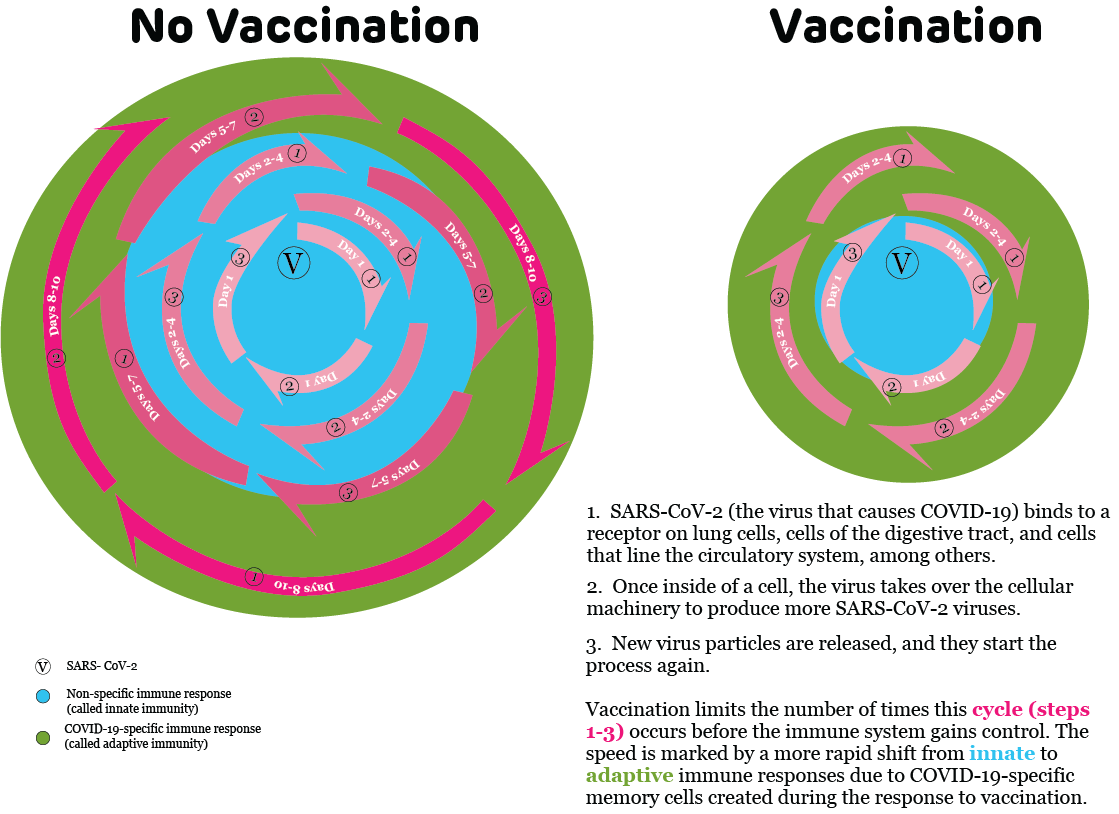
Hopefully, you were able to tell that vaccination causes changes in our immune system’s response to a virus. But because often nothing noticeable happens when vaccinated people are exposed to a virus, sometimes it is difficult to know that vaccination made a difference. So, let’s consider the different immune system reactions of two people exposed to SARS-CoV-2, the virus that causes COVID-19:
- Mr. Smith has not yet received his COVID-19 vaccine.
- Ms. Jones received both recommended doses of the COVID-19 vaccine. She finished her last dose one month ago.
Mr. Smith and Ms. Jones are teachers at the same school. After a recent staff meeting, they find out that they were both exposed to COVID-19 when another teacher did not realize he was infected.
Watch this short animation as a reminder of how a virus infects cells.
Mr. Smith’s immune system response
(represented by the “No vaccination” part of the diagram)
When the virus attaches to Mr. Smith’s cells and starts reproducing, new virus particles are released from infected cells. These cells infect nearby cells to start the process over again. In the diagram above, these repetitive cycles of viral replication are demonstrated by the pink arrows. The increasing pink color shows that as these cycles repeat, more viral particles are available to continue the process. This increasing amount of virus makes it more difficult for Mr. Smith’s immune system to stop the infection.
In the early days of the infection, Mr. Smith’s innate immune response will be in charge because his body has not encountered the SARS-CoV-2 virus previously. This is shown in the blue circle in the diagram. It will take about 5 to 7 days before the innate immune response starts to get significant help from the adaptive immune system.
The important difference between the innate and adaptive immune response is the method by which they respond to an infection. Innate immunity is non-specific; it is meant to be a first line of defense. On the other hand, adaptive immunity is specific, meaning its components have special training. In this case, adaptive immune system responses are targeted against the SARS-CoV-2 virus. As demonstrated by the green circle in the diagram, once the adaptive immune response starts, the immune system can more quickly control the infection.
After about a week to ten days, Mr. Smith’s immune system gains control, and he feels better. Unfortunately, Mr. Smith’s wife is now feeling “under the weather,” even though Mr. Smith thought he did a good job of staying isolated while he was sick.
Ms. Jones’ immune system response
(represented by the “Vaccination” part of the diagram)
It is possible that Ms. Jones’ immune system will recognize the SARS-CoV-2 virus before it can get into any of her cells and begin replicating. If this happens, she will not have any symptoms and she will not be capable of spreading the virus to anyone else. But it is also possible that a few viral particles could still replicate before Ms. Jones’ immune system realizes that she has been exposed. As in Mr. Smith’s case, the pink arrows indicate viral replication. You can see that virus does not have as much opportunity to replicate in Ms. Jones’ cells.
This is because Ms. Jones has been vaccinated. The vaccination allowed her immune system to train specialized forces to recognize the SARS-CoV-2 virus. As a result, her adaptive immune response (represented by the green circle) is able to jump into action much more quickly (within 1 to 3 days) than Mr. Smith’s did. The result is that the innate immune response (represented by the blue circle) is in charge for much less time, and Ms. Jones’ infection is shut down much more quickly. If Ms. Jones feels sick at all, she will have fewer symptoms for fewer days.
Think about it:
- What differences did vaccination make for Ms. Jones' experience?
- If Mr. Smith is exposed to the SARS-CoV-2 virus in the future, what do you think his diagram would look like? Why?
- Watch the animation, "The Innate and Adaptive Immune Systems," and think about how the information in the animation relates to the diagram and the experiences of Mr. Smith and Ms. Jones, Be prepared to share your observations in a class discussion.
February 1, 2021
Why are they having trouble getting the vaccine to people who want it?
Getting COVID-19 vaccines to the public has been challenging in the early days after their approval, so let’s consider a few reasons why.
The vaccines
All vaccines must be transported and stored in a way that maintains their effectiveness.
Both COVID-19 mRNA vaccines are temperature sensitive:
- The Pfizer vaccine must be stored at -70 degrees Celsius (°C), which is much colder than the freezers that we use in our houses. In fact, this is even colder than the average temperature of central Antarctica.
- The Moderna vaccine can be stored at “warmer” temperatures of about -20°C.
In addition to the temperature requirements, once these vaccines are thawed, they cannot be frozen again. And, once doses are taken out of the vial, the remaining doses must be used within a few hours.
These requirements mean that a lot of planning needs to go into vaccine transport, storage and use.
The supply
The number of people who want to get the COVID-19 vaccine is extremely high. And, in order to stop transmission of this virus in our communities, millions and millions of people will need to be vaccinated.
On the other hand, COVID-19 vaccines are new. Because of the pandemic, production of the vaccines was allowed to start during the clinical trials to save time. If the vaccines did not work or were not safe, they would have been thrown away. Luckily, that did not happen with the mRNA vaccines. But, even with the faster production schedule, it will still take time to make enough doses of these vaccines to immunize the majority of people in this country and around the world.
In sum, the number of people who want the vaccine combined with the fact that these are new vaccines creates a big gap between supply and demand.
The situation
Everything is more difficult in a pandemic. We cannot do things in the way we would typically do them. For example, normally when people want something, they wait in line for it. But, because of social distancing to try to slow the spread of the virus, this is not an option. And, some of the things that might be done in the summer, like moving people outside and spreading them out, are not possible when the weather is cold and unpredictable. Even though there are some ways to work around these issues, the fact remains that the risk of spreading COVID-19 itself makes organizing vaccine clinics more challenging.
The distribution plan
One of the biggest challenges has been that there has not been one centralized plan for administering vaccines. Plans for distributing the vaccine vary from state to state and sometimes even from county to county. While this makes sense in some ways because different places have different situations, a single general approach that varies in minor ways would be less confusing to the public and easier for those in charge of distribution.
Another aspect of the distribution plan is its sheer size. Millions and millions of people are waiting to get a COVID-19 vaccine. People working on distribution are trying to make sure not only that a lot of people get vaccinated, but also that the vaccine distribution is equitable for people with different backgrounds and situations all over the country. In an attempt to address this, the Centers for Disease Control and Prevention (CDC) made recommendations regarding vaccine prioritization.
While the CDC recommendations offer guidelines, it is up to each state to decide how to allocate the vaccines they receive. So, people living in one state might have different eligibility and prioritization schedules than family and friends living in another state.
Summary
Just because something is challenging doesn’t mean that it can’t be done. But it does mean that it will take hard work, creativity, and some patience.
Think about it:
- If you were in charge of COVID-19 vaccine delivery for your town/city, how would you do it?
a. How would you decide who gets the limited doses?
b. How would you have people schedule their appointments?
c. Where would you hold vaccine clinics?
d. How would you set up the clinics to keep everyone safe and ensure that you
have enough people, so that you don’t waste any doses of vaccine? - What are some of the advantages of having local areas organize their own vaccine administration? What are some disadvantages?
- If someone gave you the chance to help decide who would get immunized first, would you want to be part of that team?
- Choose 2 state health department websites and review the COVID-19 vaccine distribution information. How are their approaches similar? How are they different?
Related resources:
When Vaccine is Limited, Who Should Get Vaccinated First?, CDC
Pfizer-BioNTech COVID-19 Vaccine Storage and Handling Summary, CDC
Moderna COVID-19 Vaccine Storage and Handling Summary, CDC
January 14, 2021
What are the side effects of the COVID-19 mRNA vaccines?
- Side effect: An effect that occurs after receipt of a medication or vaccine. Even though these are unwanted, they can be expected based on previous experience (e.g., clinical trials) and result despite proper use of the medication or vaccine.
- Adverse effect: An unexpected effect that occurs after receipt of a medication or vaccine, which may or may not be caused by the medication or vaccine.
Watch this short video to learn more about the differences between side effects and adverse effects.
Side effects after COVID-19 mRNA vaccines
People are sometimes surprised when they have a side effect following a vaccine or medication, and they may perceive these effects as problematic. But, side effects do not mean there is a problem. For example, the two new mRNA COVID-19 vaccines have resulted in side effects, such as:
- sore arm (at injection site)
- tiredness
- headache
- muscle or joint aches
- fever or chills
These side effects typically occur in the first few days after receiving the vaccine, and in the case of both new mRNA COVID-19 vaccines, the side effects were more likely after the second dose. Further, younger people were more likely to experience side effects than older people. So, why does this happen and what does it mean related to the COVID-19 mRNA vaccines?
- In some cases, side effects indicate that your immune system is working to defend against the vaccine. Side effects are often part of the immune response generated by our bodies. For example, aches and pains result from changes that we can’t see, like swelling, as immune system cells move into the area where a “foreign invader” has been identified. Redness can result from increased blood flow to the area of the immune response, and fever is the body “turning up the temperature” to increase the effectiveness of the immune system and decrease the effectiveness of a perceived pathogen. As you might have realized, many side effects are things we describe as symptoms when we are sick, but in that case, too, it is our immune system responding to a “foreign invader.”
- Side effects occur in the first day or two after the vaccine because the immune system is working. After a vaccine is administered, it takes several hours for the immune response to “ramp up” and respond to what it perceives as a “foreign invader.” Often, the first effects will be closest to the injection site, like pain and redness at the site of the injection, with later effects being further from the injection site or widespread, like fever.
- The greater number of side effects after the second dose and among younger recipients suggest that mRNA vaccines invoke strong immune responses. So, even though side effects might make you “feel sick,” you aren’t actually infected. Your immune system is just responding to the vaccine. Like a fire drill, the COVID-19 vaccine trains your body to protect you from SARS-CoV-2 if you are exposed to it in the future.
Adverse events after COVID-19 mRNA vaccines
So far, one adverse event following COVID-19 mRNA vaccines has been identified. Specifically, a small number of people have had severe allergic reactions, called anaphylaxis. Currently, it is estimated that this severe allergic reaction has occurred in about 11 of every 1 million people who got the vaccine. Because these types of allergic reactions tend to occur very quickly after someone gets the vaccine, people are being advised to stay at the site where the injection was given for 15 to 30 minutes after getting vaccinated. No one has died from this reaction. Likewise, scientists and public health experts have systems in place to learn more about these reactions as well as monitor for other adverse events on an ongoing basis.
Think about it:
- Do you think some people might confuse side effects with actual illness? Why or why not?
- Why do you think some people experience side effects, but others do not?
- If a person experienced a physical condition in the days after receiving a vaccine, like indigestion or a racing heart, do you think they might believe the vaccine was the cause even if the evidence proved otherwise? Why or why not?
Related resources
Unit 1, Lesson 2 – The Innate Immune Response
What to Expect after Getting a COVID-19 Vaccine, CDC
Side Effects and COVID-19 Vaccines: What to Expect, John Hopkins Bloomberg School of Public Health
December 22, 2020
How do we know COVID-19 vaccines are safe?
Even though COVID-19 vaccines were created faster than other vaccines, their safety has not been compromised. So, how do we know?
To start, let’s look at this short video from the Centers for Disease Control and Prevention, The Journey of Your Child’s Vaccine, to see how vaccines are normally made.
So, now, let’s take a look at how COVID-19 vaccines were made using the 3 parts of the journey described in the video.
Part 1: Development, approval, and manufacture of a vaccine (purple part of the infographic)
For COVID-19 vaccines, a few changes were made to shorten the process of making the vaccines. Importantly, these changes did not sacrifice measures of vaccine safety.
- Change #1: Phases 1 and 2 of clinical trials were combined, so that the timeline could be shorter.
- Change #2: Vaccine batches (lots) were made and tested while phases 1, 2 and 3 of clinical trials were being completed. Normally, this work would not happen until after the Food and Drug Administration (FDA) licensed the vaccine. But, by doing these steps in parallel for COVID-19 vaccines, as soon as the FDA approved a vaccine, doses were ready to ship. If the vaccine was not approved by the FDA, these doses would have been thrown away.
One important thing that did not change was the size of the phase 3 trials for COVID-19 vaccines, which included tens of thousands of participants. For example, the Pfizer phase 3 trial included about 38,000 people, and the Moderna phase 3 trial included more than 30,000 people. Trials of this size may not reveal rare adverse effects, but they are large enough to find major safety issues.
Another important thing that did not change during COVID-19 vaccine development was the review process. When the data are submitted to the FDA, a special committee, called the Vaccine and Related Biologics Product Advisory Committee (VRBPAC), a group of independent experts, study the data to understand how safe and effective the vaccine is. They share this understanding with the FDA to help the FDA make their decision about whether to accept or reject the vaccine.
Part 2: Adding a vaccine to the immunization schedule (orange part of the infographic)
COVID-19 vaccines are not being added to the childhood immunization schedule, but they still go through this part of the process after the FDA’s approval process is finished. During this phase, another group of experts reviews the data. They are part of the Advisory Committee on Immunization Practices (ACIP). This committee offers guidance to the Centers for Disease Control and Prevention (CDC). They review the data and make recommendations about who should or should not get the vaccine. Even for a vaccine that will only be given to adults at first, like the COVID-19 vaccine, this committee still considers questions like:
- How safe is the vaccine for people of different ages?
- How well does the vaccine work in different age groups of people?
- How serious is the disease in different groups of people?
- How many people would get sick without the vaccine?
The CDC considers the insights offered by the ACIP to create “recommendations,” which are the rules that doctors and other healthcare providers use to decide who should or should not get the vaccine.
Since COVID-19 vaccines will eventually also be given to children, this committee will meet again to make rules related to the use of these vaccines in children.
The ACIP usually meets three times per year, and new vaccines are added to the agenda at that time. For COVID-19, as soon as the FDA approved each vaccine, the CDC held emergency meetings a few days later to review the data and make recommendations. In this way, the timeline was also shortened because the companies did not need to wait until the next scheduled meeting. (For more information about the CDC’s part of the process during COVID-19, check the “Related Resources” section for a link to the video, How CDC is making COVID-19 vaccine recommendations.)
Part 3: Continuing to monitor vaccine safety (green part of the infographic)
By the time a vaccine can be given to the public, the data from phase 3 trials have been reviewed by dozens of independent experts, but that does not mean that the FDA and the CDC are done monitoring the vaccine. As described in the video and shown on the infographic, several different systems monitor vaccine safety:
- Vaccine Adverse Events Reporting System (VAERS)
- Vaccine Safety Datalink (VSD)
- Post-licensure Rapid Immunization Monitoring System (PRISM)
- Clinical Immunization Safety Assessment Project (CISA)
These systems work in different ways, so that the FDA and the CDC have many chances to look for vaccine safety concerns.
For COVID-19 vaccines, the CDC will use two extra monitoring systems:
- V-safe – This new smartphone-based monitoring system allows the CDC to communicate with people who recently got the COVID-19 vaccine through texts and web surveys to see how they are feeling.
- National Healthcare Safety Network (NHSN) – This system is not new, but it will help monitor COVID-19 vaccinations at long-term care facilities, like nursing homes.
Summary
- Changes to part 1 made the process shorter, but did not delete any part of the process.
- The same was true for part 2.
- Part 3 is always ongoing for any vaccine, but extra monitoring has been added for COVID-19 vaccines.
Think about it:
- After learning about the changes for COVID-19 vaccines, which changes do you think were most helpful for quickly creating a vaccine?
- Were there changes that you feel should not have been made?
- As a class, discuss the ethics of vaccine development during a pandemic. On one hand, a vaccine is needed as soon as possible so fewer people die from the disease. But, on the other hand, vaccine safety is even more important because the vaccine will be given to millions of people in a short period of time.
- Many people are concerned about the safety of COVID-19 vaccines because of the short amount of time in which they were made. What do you think is the most important thing for these people to know? If you worked at the CDC, how would you get this information to people? For example, what message would you create and where would you share it?
Related resources:
The Journey of Your Child’s Vaccine (Video), CDC
The Journey of Your Child’s Vaccine (Infographic), CDC
Ensuring the Safety of COVID-19 Vaccines in the United States, CDC
COVID-19 Vaccines, FDA
Emergency Use Authorization for Vaccines Explained, FDA
How CDC is Making COVID-19 Vaccine Recommendations (Video), CDC
December 9, 2020
Can you explain how mRNA vaccines work?
Messenger RNA, or mRNA, is a nucleic acid that is common in our bodies. It is the link between DNA, our genetic code, and our body’s ability to make proteins. In short, mRNA is made from DNA in the nucleus of cells. The mRNA is then transported to the cytoplasm, where it serves as the blueprint during protein manufacture. So, how can it be used to help us make a vaccine?
With mRNA vaccines, instead of introducing a weakened or inactivated pathogen, like other vaccines, mRNA is introduced as the vaccine. The mRNA that is chosen represents a part of the virus to which the body would make an immune response during an infection. In the case of COVID-19, the mRNA is against the SARS-CoV-2 spike protein. As cells generate and release the protein, the immune system recognizes the protein as “foreign” and works to get rid of it, generating an immune response.
mRNA vaccine technology has been studied in animals for many years, but COVID-19 mRNA vaccines are the first to be approved for use in people.
Watch a short video in which Dr. Offit discusses how mRNA vaccines work.
Related resources:
From DNA to Protein (Animation), Wellcome Genome Campus for yourgenome.org
Understanding mRNA COVID-19 Vaccines, CDC
September 30, 2020
Why do we need to wear masks, and what do we need to know about them?
SARS CoV-2, the virus that causes COVID-19 disease, is a respiratory disease, so it can easily spread from one person to another through the air by coughing, sneezing, or even talking. Since the virus particles are in the secretions from our noses and mouths, masks that cover both the nose and mouth serve as a physical barrier to the spread of the virus particles. In fact, a number of scientific studies have proven that masks can help decrease the spread of SARS CoV-2 virus when worn properly.
Masks are most likely to reduce the spread of COVID-19 when most people in public settings use them. The Centers for Disease Control and Prevention (CDC) recommends that people wear a mask in public and whenever they are around people who do not live in their household. People taking care of someone in their home who is ill with COVID-19 should also wear a mask. Masks help in two ways:
- If you are infected, even without symptoms, you have a lower chance of spreading the virus to others.
- If virus particles are in the air, because someone near you is infected, you have a lower chance for virus particles to enter your nose or mouth as you breathe.
These measures are particularly important because SARS-CoV-2 can spread even when people do not have symptoms of COVID-19. Scientists estimate that up to 4 of every 10 people infected with SARS-CoV-2 do not have any symptoms, so they can spread the virus and never know that they did it.
Think about it:
Can you name any other viruses that might be stopped by masks? Why do you think masks are not recommended to stop those viruses?
Proper mask wearing
Did you know there are steps to follow when you are putting on a mask or taking one off?
Putting on protective equipment, like a mask, is called “donning.” Proper mask donning involves these steps:
- Wash your hands or use hand sanitizer that contains at least 70% alcohol to make sure your hands are clean before you touch the mask.
- Put the mask over both your mouth and nose, and make sure it is secure under your chin without any gaps.
- Make sure the mask fits snugly around your nose. If it has a metal band at the top, shape it so that air is not escaping through the top of your mask.
- Check to see that the mask fits snugly on both sides of your face without any gaps.
- Make sure you can breathe and talk easily with the mask in place.
While wearing your mask:
- Try not to touch the mask while you are wearing it. If you do touch the mask, either wash your hands with soap and water or use hand sanitizer containing at least 70% alcohol to disinfect your hands.
- Be sure to wear your mask properly. Look at the examples below to see correct and incorrect ways to wear your mask:
Correct
Incorrect
Taking off protective equipment, like a mask, is called “doffing.” Proper mask doffing involves these steps:
- Whenever you are removing or handling your mask, be careful not to touch your mouth, eyes, or nose.
- Only touch the mask by the ear loops or the tie behind your head. This is to avoid any germs that are on the mask itself.
- Loosen the band around the ears or untie the band behind your head.
- If you will be discarding the mask: Fold the outside corners of the mask together, so the part that was facing “the world” is on the inside. That helps keep any virus particles that could be on the mask away from your hands.
- If you will need to use the mask again: Sometimes if you are at school or at a restaurant, you will need to take your mask off for a short time and then use it again. In these cases, fold the outside corners of the mask together, so that the part that was touching your face is on the inside. That way, when you put the mask on again, the “inside” of your mask will not have touched any surface that could be contaminated with the virus. Try to place your mask on a clean surface or in a place where it will not be disturbed until you need it again.
- If the mask is disposable, throw it in a trash receptacle. If it is washable, put it in the laundry. When it is washed, soap and hot water should be used, and it should be dried in the dryer.
- Wash your hands immediately after you remove the mask and place it in the trash or laundry receptacle.
Doctors, nurses, and other healthcare providers are used to donning and doffing protective equipment because they have to do it all the time to protect themselves and their patients.
Types of masks
Masks should be worn when interacting with others, especially anyone who does not live in your home, and masks should not be shared with others. Everyone should have their own. Children under 2 years of age and people who have trouble breathing with a mask or who could not remove the mask without help should not wear masks. In these situations other precautions, like hand washing and social distancing, are even more important, as is the need for those around these individuals to wear masks.
Several types of masks are effective against COVID-19. A study conducted at Duke University showed that the best masks include three-layer surgical masks as well as those with 2 layers of cotton material. Homemade masks are effective as long as they are made of 2 layers of cotton. Another study from the University of Arizona showed that masks made from tea towels, cotton-blended fabrics, and antimicrobial pillowcases were protective. The authors reported that the more densely that the fibers are packed in a material, the better it is at filtering, and, therefore, the better it will be as a mask. So, for example, materials with higher thread counts are more protective.
Several types of masks have been found not to work very well. For example, handkerchiefs or bandanas, neck gaiters, and knitted masks provided little protection against the virus in the Duke University study. The materials that were least effective at blocking the virus in the University of Arizona study were scarves and those made from cotton t-shirts.
Other masks, including N-95 and N-99 masks, are very effective against the virus, but due to their short supply, they are reserved for health care personnel.
- Check out the types of masks tested in the study from Duke University.
- Check out the types of masks tested in the study from the University of Arizona.
Think about it:
These two studies both aimed to learn about what types of masks are most effective, but they were conducted in different ways. After reviewing them, think about whether one method seems better than the other. Why? Why do you think it might be important for studies answering similar questions to be done in different ways? What benefits might this provide? What drawbacks might there be?
Related resources
How to Wear a Mask, CDC
Coronavirus Face Masks & Protection FAQs, Johns Hopkins
Q&A: Masks and COVID-19, World Health Organization (WHO)
WHO’s recommended fabric mask materials and composition, WHO
May 19, 2020
What is "contact tracing?"
Contact tracing is a pillar of infectious disease control in public health. It is a well-tested technique for controlling diseases that spread from person-to-person. In fact, contact tracing was central to the eradication of smallpox. Public health officials used exhaustive contact tracing to find all infected individuals. By isolating infected persons and immunizing contacts, and members of the surrounding community, the spread of smallpox was completely stopped.
So, how does contact tracing work?
The basics of contact tracing
When public health officials identify infected people, they interview them about whom they had recently contacted. For COVID-19, this means identifying who people had contacted 2 days before symptoms started through the time when they started isolating.
Knowing these people, called “contacts,” may have been exposed to the disease, officials reach out to alert them of possible exposure and collect information from them. In this manner, contacts can be tested, isolated, and treated if infected. Likewise, their contacts can also be identified – essentially, the second generation of contacts.
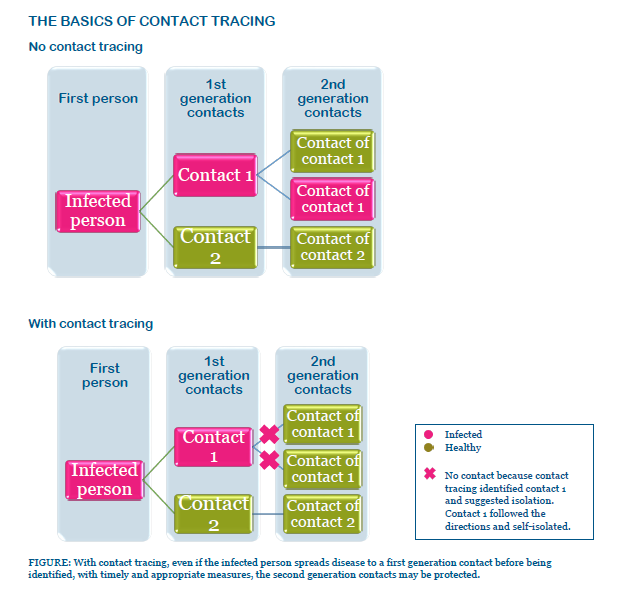
Goals and timing of contact tracing
Contact tracing can reduce the spread of infections in a community by:
- Interrupting person-to-person transmission
- Offering diagnosis, counseling and treatment to individuals who are already infected
- Alerting contacts that they might have been infected and providing counseling or care to decrease the chance of becoming sick
- Learning more about how the disease moves through communities
In contact tracing, time is of the essence. The longer it takes to identify contacts, the more people they will be in contact with. Ensuring that contacts do not interact with others is critical to protecting communities from further spread. Whereas people with influenza infect an average of two additional people, those infected with COVID-19 are estimated to infect about six additional people when doing day-to-day activities in the community. If communities are not able to effectively isolate infected patients and ensure that contacts can separate themselves from others, diseases can rapidly spread.
Contact tracing and COVID-19
Contact tracing is an important part of the current COVID-19 pandemic for a few reasons:
- Almost everyone is susceptible to infection.
- We don’t yet have effective prevention measures (e.g., vaccines).
- We are still learning what testing results mean.
- We don’t have many effective treatments yet.
A person is identified as a contact of a COVID-19-infected person if they have been within 6 feet of that person for a period of at least 15 minutes without wearing personal protective equipment.
Based on what we know about the virus at this point, contacts of people infected with COVID-19 are encouraged to stay home for 14 days after their last interaction with the infected person and maintain social distance — at least 6 feet — from others.
Identified contacts are also instructed to:
- Monitor themselves for symptoms, like cough or shortness of breath
- Check their temperature twice daily
Sometimes public health staff will check in with identified contacts to make sure they are self-monitoring and have not developed symptoms. Typically, once contacts have been identified, they will be given information about how to get in touch with the health department. If a contact develops symptoms, they will be evaluated for infection and need for medical care.
Because contact tracing is one of the best tools we have to stop the spread of COVID-19 at this point, it is critical that anyone identified as infected, or a contact of someone who is infected, follow the instructions they are provided.
May 12, 2020
What is a "cytokine storm?"
Some patients with COVID-19 have been reported as experiencing an overreaction of their immune system, referred to as a “cytokine storm.”
Cytokines are chemical signals that enable communication between parts of the immune system, triggering specific cells to respond to a pathogen.
Cytokine storms are not unique to COVID-19; they can occur during other infections as well.
Find out more:
- Cytokines – See “Preparing for battle” in the “Adaptive immune system” section of this page.
- General information about cytokine storm – See bottom of the section titled, “Unnecessary or overzealous immune responses” on this page.
- COVID-19 cytokine storm and research occurring at CHOP – “Multidisciplinary Team Studies Cytokine Storms Brewing in COVID-19,” by Jillian Rose Lim, CHOP Research Institute Cornerstone Blog.
April 23, 2020
Can you explain COVID-19 testing?
We have been hearing a lot about testing during the coronavirus pandemic, so let’s take a closer look. Discussion about testing related to COVID-19 refer to two different types of testing – infection testing and antibody testing. Infection testing measures the presence of virus in a person’s respiratory secretions. In contrast, antibody testing, as it sounds, measures the immune response after an infection by testing for antibodies that a person has against the virus. Antibody tests require blood samples instead of respiratory secretions.
Infection testing
This is the first kind of test we heard about when the pandemic started, and it continues to be important. Infection testing tells whether a person is currently infected with the virus. Most of these tests involve swabbing the nasal cavity to collect respiratory secretions that may contain the virus.
These samples are processed using a lab technique called reverse transcriptase polymerase chain reaction, or RT-PCR. Lab technicians isolate coronavirus RNA from the nasal sample and using the enzyme, reverse transcriptase, they create “complementary DNA,” or cDNA. The cDNA is like a model, or pattern, that can be used to make copies of DNA that mimic a portion of the coronavirus RNA. To do this, technicians add a few other reagents to the sample before placing it in a piece of lab equipment, called a PCR machine, which is programmed to the conditions needed to build DNA. The other reagents include:
- A piece of DNA that matches the part of the cDNA that they wish to amplify, or copy. This is called a primer.
- Deoxyribonucleotide triphosphates or dNTPs, which are the building blocks to make more DNA. These are more specifically known as dATP (adenine), dGTP (guanine), dTTP (thymine) and dCTP (cytosine).
- An enzyme that will allow for more DNA to be produced, called DNA polymerase.
- One other important agent that is added to the PCR sample is a fluorescent marker that enables scientists to determine whether the sample is positive or negative.
If the patient sample had coronavirus RNA, at the end of the PCR process, the sample will have many copies of DNA that contain the fluorescent markers. If the sample did not have coronavirus RNA, the sample will not have new copies of DNA, and, therefore, will not fluoresce. Fluorescent markers can be detected in a few ways:
- In some labs, the PCR machine is connected to a computer and includes a component that can detect fluorescence. This is called “real-time RT-PCR” because scientists can monitor fluorescence as the PCR reaction occurs. This approach results in safer and faster processing of samples.
- However, in many cases, the PCR samples are subjected to a second assay, called an enzyme-linked immunoassay or ELISA. In this method, rather than using fluorescent markers, other molecules will be used as markers. During the ELISA, the molecular markers will be detected by specially labeled antibodies that bind to them. Through a series of additional steps, the labeled antibodies will be detectable by a color-based reaction that is analyzed using a special piece of equipment made for reading these color changes in ELISA samples.
In the case of the current pandemic, the important result is qualitative, meaning knowing whether the sample was positive or negative. However, in other situations RT-PCR can be used to generate quantitative information, meaning that scientists can measure differences between the amount of RNA or DNA in the starting samples.
Infection testing will remain important for determining when individuals are infected with COVID-19, so this type of testing will continue to be used to diagnose patients.
Watch this short video to hear how scientists and clinicians at CHOP developed a COVID-19 infection test.
Antibody testing
More recently, we have been hearing about antibody testing. Antibody tests are important for a few reasons:
- They can determine who was infected previously, including people who did not feel sick or have symptoms, called asymptomatic.
- They can be used to determine who can be a plasma donor for the treatment approach currently being tested to help coronavirus patients recover.
- They will be critical for learning more about who is protected from infection, particularly during vaccine trials.
While this testing is essential, it is important to realize that right now, we are still learning about this new virus, so it will take time for scientists to understand exactly what antibody test results mean. Specifically, we need to understand:
- When was someone infected, and how long will their antibodies last? Right now, we do not know how long antibodies will remain after coronavirus infection. Typically, when someone has an infection, antibody levels increase in the first week or two after exposure, and after recovery, the levels decrease over time. For most infections, even if antibodies are not detectable with a clinical test, the person still has immunologic memory. With immunologic memory, if the person is exposed again, their immune system can respond more quickly. Likewise, the antibodies generated from memory cells are more adept at stopping infection than those generated during primary, or first, infection. Based on historical studies with human coronaviruses, it is expected that immunologic memory generated after infection will protect people from having moderate or severe disease, even if they are infected again, but this needs to be confirmed for COVID-19 virus.
- What type and quantity of antibodies are needed to prevent infection? Right now, we also do not know what type of antibodies (See “Adaptive immune system” on this page of the VEC website for a description of antibody types) will be most effective at preventing infection and how high the level of antibodies needs to be for protection. In addition to better understanding the different types of antibodies, it is also important to make sure that the antibodies are capable of neutralizing the virus, not just binding to it. This means scientists need to understand how the antibodies function. Typically, when making a vaccine, scientists want a “marker for efficacy,” meaning some measurement that indicates the vaccine works well enough to prevent people from getting sick if they are exposed to the virus (i.e., generates sufficient quantities of antibodies as well as antibodies capable of neutralizing the virus). Because COVID-19 is a new virus, scientists do not have enough experience yet to have defined this type of marker.
Two additional challenges to the current antibody testing situation involve the development of early tests. First, because of the emergent nature of the situation, the Food and Drug Administration (FDA) is allowing some tests to be used before the typical FDA reviews have been completed. So, some tests will ultimately not meet with FDA standards. Antibody tests need to be both sensitive and specific:
- Sensitivity means the test needs to accurately detect positives, or, said another way, it needs to accurately detect who had disease.
- Specificity means that the test should not result in false positives; that is, it should not give a positive result when the person does not have coronavirus antibodies.
Scientists aim for tests that accurately measure sensitivity and specificity about 99 of 100 times, essentially only missing 1 positive person and only falsely identifying 1 person as positive, when they are actually negative. Because the tests are not all reviewed before being available, we do not know the sensitivity and specificity of all tests. A small study of several tests found a wide range of sensitivities and specificities (Lassaunière, 2020).
Second, scientists still need to figure out the timeline related to infection and development of the immune response to understand the best time to use antibody tests. For example, someone may have detectable antibodies, suggesting that they had an infection, but they may also still be infectious. In other words, they could have both a positive infection test and a positive antibody test. The relative timing related to the presence of virus in a person’s secretions and the detection of antibodies in their blood still needs to be better understood, especially if politicians aim to use antibody testing data as a means for deciding when to reopen communities.
Two types of antibody tests are being developed – lab-processed and point-of-care tests:
- Lab-processed tests require sending the blood sample to a clinical laboratory, where the samples are subjected to an ELISA assay. These blood samples are collected by insertion of a needle into the patient’s arm. The ELISA assays used to process blood samples are similar to those mentioned as part of the PCR process in the “infection testing” section (above) in that they employ antibodies and color-change-based reactions. However, they use different reagents, so that the results measure COVID-19-specific antibodies in a person’s blood (rather than the DNA generated during PCR). Some of the lab-processed tests being developed measure any COVID-19 antibodies, but others measure specific sub-groups of antibodies, like IgG or IgA. (See “Adaptive immune system” on this page of the VEC website for a description of antibody types.)
- Point-of-care tests are processed at the time the blood is taken from the patient. These are the type that have been shown being used at drive-through testing sites on the news. Samples are typically obtained by a prick of the finger, and the blood wicks into a narrow tube before immediately being added to a small cassette, which is about the size of a microscope slide. Within 10-15 minutes, the results show whether a person has COVID-19 antibodies. Typically, these tests measure IgM or IgG antibodies.
While point-of-care tests are faster and easier to process, they can only determine positive or negative results (qualitative) and may prove to be less accurate.
The conundrum that we currently face is that to develop policy, large portions of the population will need to be tested, so a faster antibody test that requires less blood, such as the point-of-care tests offer, would be much more conducive to getting a large amount of information quickly. However, results from these tests need to interpreted with caution until scientists can determine their accuracy and limitations. Likewise, since several different point-of-care tests are available, and since their quality is likely to vary, comparing data generated from areas using different tests is also likely to be problematic.
Both infection and antibody tests will continue to be necessary in controlling COVID-19.
Selected references and resources
- Daly, J. 2020 Mar 27. Here’s how coronavirus tests work – and who offers them. Scientific American. https://www.scientificamerican.com/article/heres-how-coronavirus-tests-work-and-who-offers-them/
- Lassaunière R, Frische A, Harboe ZB, et. al. 2020. Evaluation of nine commercial SARS-CoV-2 immunoassays. MedRxiv preprint doi: https://doi.org/10.1101/2020.04.09.20056325.
- Mallapaty, S. 2020 April 18. Will antibody tests for the coronavirus really change everything? Nature. https://www.nature.com/articles/d41586-020-01115-z
April 14, 2020
What are the similarities and differences between influenza and novel coronavirus?
There have been some questions about whether influenza and coronavirus are similar. So, let’s take a closer look.
The viruses
While influenza and COVID-19 are both enveloped viruses that contain RNA, they are not related. Influenza viruses are from the family Orthomyxoviridae, whereas coronaviruses are from the family Coronaviridae. See more details:
- Both have envelopes. Viral envelopes are made of lipids, which are a type of fat molecule that cannot dissolve in water. However, these molecules are easily damaged by disinfectants, which is why soaps, alcohol-based hand sanitizers, and many cleaning agents can reduce the chance of infection with both.
- Both have protein spikes that help them attach to cells. Influenza viruses have two surface proteins, called hemagglutinin and neuraminidase. These two spike proteins are commonly used to distinguish among different influenza viruses (e.g., H1N1, H2N3, etc.) The protein that causes the spikes on COVID-19 (and other coronaviruses) is generally referred to as “S protein.”
These spike proteins determine what parts of the cell surface the viruses attach to when they infect people. Influenza viruses bind to sialic acid, whereas coronaviruses bind to an enzyme receptor, commonly referred to as ACE-2. Preventing binding of the virus to the cell surface is one way to prevent infection, so this information is important for the development of treatments and vaccines.
- Both contain RNA. Influenza viruses and coronaviruses have nucleic acids that are single-strands of RNA. In contrast, human cells contain double-stranded DNA. Like individual strands of DNA, RNA is labelled as “positive” or “negative.” These labels relate to how the strands are used during replication. Positive strands of RNA can be used as a roadmap during protein production (translation), but negative strands must first be made into a template (transcription) before proteins can be produced. Coronaviruses contain positive strand RNA, whereas influenza viruses have negative strand RNA.
Another difference between the RNA contained in these two types of viruses is how it is contained in the cell. The RNA in coronaviruses is one long strand; this is called a non-segmented genome. In contrast, the RNA in influenza viruses is segmented, meaning it is in several pieces. Influenza type A, which is the type that can cause pandemics, contains eight segments of RNA, as do influenza type B viruses. Other types of influenza viruses, called type C and type D, have seven RNA segments.
- Both can infect animals and people. One of the reasons viruses from both of these families can cause pandemics is because they can change rapidly when they “jump” from animals to people. Typically, the types of a virus that infect people are less capable of infecting animals and vice versa. So, for example, a human influenza virus would not typically infect a chicken. However, on occasion, genetic changes enhance the ability of an animal virus to infect people. If this genetically altered virus can also replicate efficiently and be easily transmitted from person to person, it has the ability to spread throughout the population. This is what happened with the novel coronavirus.
Although these lists may not be exhaustive, they offer a glimpse of how many different types of animals both of these families of viruses can infect:- Influenza viruses can infect birds, aquatic birds, pigs, horses, dogs, cats and sea mammals.
- Coronaviruses can infect bats, pigs, cats (domesticated and non-domesticated), dogs, rabbits, mice, rats, cows, chickens, turkeys and pangolins.
Transmission and Illness
Some of the confusion about whether these viruses are related might have come from the similar ways they are transmitted and some of the symptoms they cause.
- Both are spread by respiratory droplets. Because both of these viruses reproduce in cells that line the respiratory tract, they can be spread in respiratory secretions, such as from coughs and sneezes. Likewise, when these secretions land on objects that are subsequently touched by others, virus particles can spread to hands. If that person then touches their eyes, nose or mouth, the virus can infect them. This is why protective practices, like covering coughs, washing hands, and using masks, have been promoted during the coronavirus pandemic.
- Both can cause similar symptoms. Some people who get infected with influenza will not have any symptoms. The same is true of those with coronavirus infections. Most people, however, will have mild symptoms. Symptoms of both infections can include coughing, extreme fatigue, fever, headache, muscle aches, and runny nose or congestion. Some people with coronavirus may also have difficulty breathing, sore throats or diarrhea. More people will become severely ill with COVID-19 than what is typical with influenza virus infections. According to current data, up to 15 of every 100 people will need oxygen and about 5 of every 100 will become critically ill.
- Both are dangerous for older people and those with chronic conditions of the heart and lungs. Because both of these infections affect the lungs, those with chronic conditions of the heart or lungs are more likely to become severely ill. Likewise, others who are immune compromised, such as elderly people, are also at increased risk for complications when infected with either of these viruses. Early data suggest, however, that while pregnant women are at increased risk of severe pneumonia with influenza as compared to women of the same age who aren’t pregnant, they do not appear to be at higher risk with coronavirus.
Treatments and vaccines
While antiviral medications and vaccines are available to fight influenza, the same is not currently the case for COVID-19, since it is a new virus. However, many scientists around the globe are working to better understand which treatments will work and how to create an effective vaccine. Because these viruses are different, previous influenza infections or vaccination against influenza will not protect against infection with coronavirus. Nor, will treatment with the same medications necessarily be effective.
What will staying at home do to help with the virus, and what does flattening the curve mean?
COVID-19 can infect anyone who is not immune. People can become immune to a pathogen in two ways – through infection or vaccination. Therefore, in the absence of vaccines, the only way for people to become immune is by infection.
The issue, however, is that because COVID-19 is a new virus, billions of people lack immunity. So, if everyone continued with their daily activities, the virus would spread very rapidly through the population. While this would quickly increase immunity among the population at large, and many individuals would have mild, or non-existent, symptoms, that would not be the case for everyone. Not only would some people have severe and fatal infections, this approach would also overwhelm healthcare systems, resulting in even more deaths than necessary.
In an attempt to prevent this, public health officials have implemented social distancing to slow the spread of COVID-19. By staying apart, we will decrease the number of people with infections at any one point in time. This will cause the curve to stay lower over a longer period of time, hence the term “flattening.”
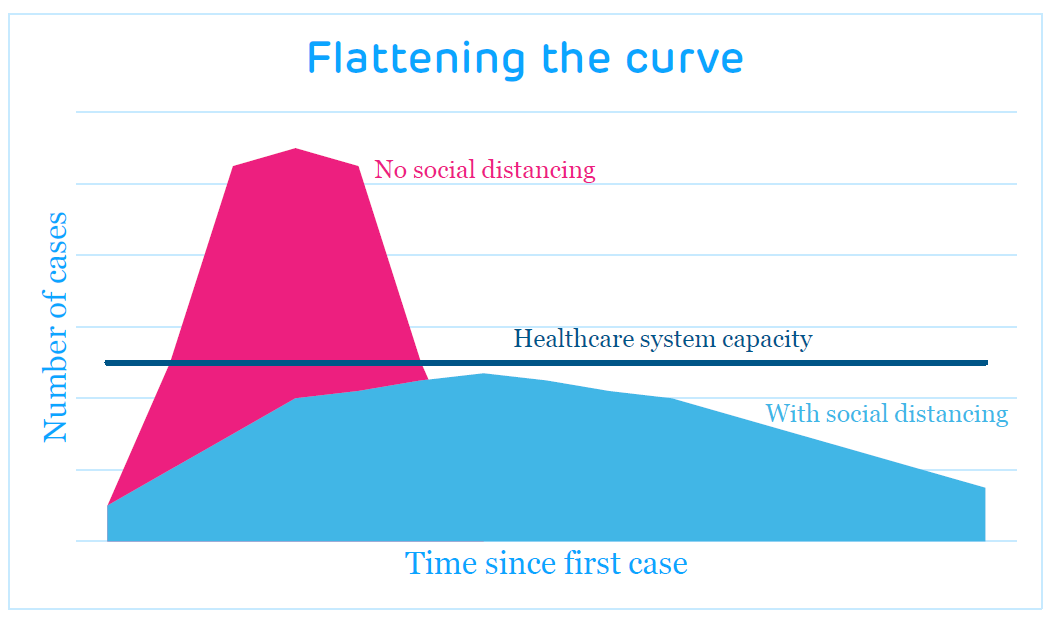
You can think of “flattening the curve” like eating at a buffet. Overfilling your plate in a single visit to the buffet will be faster, but you will lose more food than if you make several trips and fill your plate with small amounts each time. If your plate is heaping, food is more likely to be wasted when it falls off your plate on the walk to the table, when the table gets bumped, or when it mixes flavors with other foods on your plate. On the other hand, if you make multiple trips to the buffet, you will not lose as much food to the floor, table or because of mixing flavors. In the case of the pandemic, the stakes are higher because we are not losing food, we are losing family, friends and neighbors. By flattening the curve, we are giving healthcare providers a situation more akin to multiple trips to the buffet — fewer patients at once and more time to restock and regroup along the way.
This approach is also important for another reason. It lengthens the timeline for the population to gain immunity. While this might seem counter-intuitive since we want people to be protected, this approach allows scientists more time to create a vaccine. With a safe and effective vaccine, people can become immune without the chance of experiencing severe, or fatal, infections. Since we don’t know which people will get critically ill, or die, from their infections, vaccination removes the risk that is inherent in experiencing natural infection.
In sum, flattening the curve helps us in three major ways:
- Keeps the medical system from being overwhelmed
- Provides time to develop a vaccine
- Prevents more deaths
April 6, 2020
What kinds of vaccines are being studied?
One way that we hope to eventually protect ourselves from COVID-19 is by vaccination. Vaccines introduce our immune systems to a pathogen without causing disease. When people are infected with a pathogen, like the virus COVID-19, we don’t know who will become severely ill, or even die, from the infection. So, if people can become immune without getting sick, we can decrease the number of people who are hospitalized or die.
Right now, we don’t have a vaccine against COVID-19, but scientists are working to develop one. It will take several months to a couple of years to make a vaccine that has been sufficiently tested to ensure its safety and effectiveness. It will also take time to build supply, so that we have enough doses to protect large numbers of people. But, in the interim, people are, understandably, curious about how scientists are trying to make a vaccine.
Several groups of scientists are working on vaccine development, and as is typical in science, they are trying different approaches. Over time, we will see which methods work best. The approaches can be categorized into two types – those that have been used to create one or more existing vaccines and those that aren’t made in a similar manner to any current vaccines.
Previously employed approaches
Four previously successful approaches are all being examined in the effort to develop a vaccine against COVID-19:
- Inactivated vaccine – Like influenza and polio shots, this approach uses killed whole virus to make the vaccine.
- Subunit vaccine – Like hepatitis B, shingles, and HPV vaccines, this approach uses part of the pathogen to make the vaccine, like a protein or polysaccharide. The part of the pathogen chosen is that which generates immunologic protection.
- Weakened, live viral vaccine – Like measles, mumps, rubella and rotavirus vaccines, this approach involves growing the virus in cells in the lab to weaken it, so that it replicates in vaccine recipients without causing illness.
- Replicating viral vector vaccine – Like the Ebola and Dengue vaccines, this approach involves putting a gene for a protein of interest (e.g., one that will protect against disease) into a virus that will not cause illness, but will cause an immune response. These viruses are called vectors; examples of vector viruses include yellow fever vaccine virus and vesicular stomatitis virus. When the viral vector reproduces in the vaccine recipient, the protein of interest is also produced, so immunity is generated against that protein as well.
Promising new approaches
Three approaches, that are theoretically useful, but from which products have not resulted to date, include:
- DNA vaccine – Plasmids are small circular fragments of DNA. DNA vaccines are made of plasmids that have genes of interest inserted. When the plasmids are injected into muscle cells, the DNA is treated like cellular DNA, which means it is transcribed into messenger RNA (mRNA), from which proteins are produced. In this manner, the cell will produce a protein that the body realizes is foreign and creates an immune response against. Clinical trials of HIV and influenza vaccines have used this approach, but, to date, the approach has not resulted in a vaccine that is routinely used.
- mRNA vaccine – In this approach, the mRNA is the vaccine, so unlike DNA vaccines, the response does not rely on DNA being converted to mRNA, essentially cutting out a step. Like DNA vaccines, this approach has been tested in small trials, but experience with this approach is even more limited than with the DNA vaccine approach.
- Non-replicating vector vaccine – Similar to the replicating viral vectors (described above), a gene of interest is added to a vector, like vesicular stomatitis virus or yellow fever vaccine virus, and delivered to the vaccine recipient.
Finally, worth noting, one type of passive immunity is also being explored. Passive immunity is protection afforded by someone else’s pathogen-specific antibodies. Typically, we think of maternal antibodies protecting babies, but passive immunity is used in other situations as well. Called immunoglobulins, this treatment with antibodies is often used to help people after a bite by a snake or rabid animal. But, this approach was also used before we had a diphtheria vaccine and in some cases, it is used in conjunction with vaccination, such as for newborns whose mothers are infected with hepatitis B. During the coronavirus pandemic, antibodies preparations from those who have recovered are being tested for their ability to help infected patients fight the virus.
Related resources:
- VMP Middle School Lesson 4, “On the Shoulder of Heroes: Toward a World without Polio.” In this lesson, students study the scientific process as it relates to our historical understanding of polio. Students research scientists or teams of scientists to understand who discovered what, when, where, and why. Activities include creation of a class timeline and map as well as a class discussion about how the activities illustrate the scientific process of gaining new knowledge.
- Making vaccines, website section, Vaccine Education Center at Children’s Hospital of Philadelphia
- Lurie N, Saville M, Hatchett R, and Halton J. Developing Covid-19 Vaccines at Pandemic Speed, New England Journal of Medicine, March 30, 2020.
What kinds of treatments work?
Scientists and healthcare providers do not know for sure what treatments will be most effective in treating this new virus. However, they have to make treatment decisions when patients are sick. For now, they are using information about how to treat related viruses, like SARS and MERS, and as time passes, they gather more knowledge about what works, or doesn’t, in COVID-19-infected patients. Of critical importance, however, is the realization that the only way to know if a treatment works is through scientific studies.
Types of studies and ethical considerations
Information about what works can be ascertained in a few different ways:
- Placebo-controlled clinical trials compare similar patients who did and did not receive a particular treatment. With treatment and control groups, clinicians and scientists can determine whether the treatment group improved or recovered more quickly than those who did not get the treatment.
- Retrospective studies evaluate medical records of patients after treatment has finished. These studies analyze how patients responded to certain treatments, compared with other patients who did not receive the treatment.
- In some cases, study participants do not need to be individuals who are infected. For example, people who have been knowingly exposed to the virus, such as close contacts of infected patients and healthcare workers, can be treated.
Regardless of the approach, before a study can be started, it must be reviewed and approved by a committee of experts at the institution where it is being conducted. This group is known as the Institutional Review Board or IRB. These people ensure that research participants are treated ethically and safely.
Possible COVID-19 treatments
Scientists and clinicians are working quickly to find possible treatments. They will consider treatments that are already used to treat other illnesses as well as newly developed, coronavirus-specific treatments.
So, what kinds of treatments are being studied and why?
- Antiviral agents – Medications that treat viruses can act in several ways, including preventing the virus from infecting cells, decreasing its ability to replicate, or stemming immune response to the infection. Some antiviral medications are currently being tested for their effectiveness in people before or after exposure to coronavirus, like close contacts and healthcare workers, in an effort to decrease the number of infections.
- Anti-parasitic agents – One medication that has received significant attention is hydroxychloroquine. This medication is typically used to treat malaria as well as some autoimmune diseases, like lupus and rheumatoid arthritis. In March 2020, the Food and Drug Administration (FDA) approved emergency use of hydroxychloroquine for treatment of COVID-19. If effective, the medication would control the immune response to infection.
- Antibiotics – Although antibiotics are not effective against viruses, azithromycin (commonly known as Z-pack) is currently being tested for its anti-inflammatory abilities. Azithromycin together with hydroxychloroquine is being evaluated as a coordinated approach to decreasing inflammation and suppressing immune system activity to control symptoms in severely ill patients.
- Anti-inflammatory agents – In some cases, people are getting severely ill because their immune system overreacts to the infection. This type of heightened response, sometimes referred to as a “cytokine storm,” can be the cause of severe illness in young, healthy people. When this happens, medications that inhibit the overzealous response may help. One example of a class of medications being tested in these cases is called “IL-1/IL-6 antagonists.”
- Vitamin C – Much discussion has also occurred around whether vitamin C would be helpful in treating or preventing coronavirus. No evidence exists that taking vitamin C would protect someone from becoming infected with COVID-19. Some studies are currently evaluating whether vitamin C given to hospitalized patients, together with other treatments, is effective. Data are not currently available to substantiate its effectiveness.
While several of these have shown promise anecdotally, to date, insufficient evidence exists to prove that any of them effectively treat COVID-19. Hopefully, soon, evidence will be available to inform treatment decisions.
April 2, 2020
Why don’t we close down society for influenza like we are doing for coronavirus?
Some people have asked why we are acting differently about coronavirus when we don’t go to the same efforts each year when influenza season occurs. In fact, every year hundreds of thousands of people are hospitalized and thousands die from the flu. Three reasons are behind this difference:
- Biology of the viruses – Before December 2019, COVID-19 was unknown. Because it is a new virus, officials do not know some of the most basic information about it. They do not know how well the virus spreads. They do not know how long or how severe illness caused by infection can be. They do not know which people are most at risk of being infected. In contrast, influenza virus is well studied. While the virus changes frequently and, therefore, is difficult to control, we still know much more about influenza than COVID-19.
- Susceptibility –The first group of ill patients were reported in China in late December 2019. Because this virus can cause illness and it is a new virus, virtually everyone on the planet could be infected with it. On the other hand, influenza viruses circulate every year, so most people have at least some immunity to influenza.
Because virtually everyone can get sick and we don’t know as much as we need to about how to control the virus, it could spread very quickly. If too many people get sick at the same time, the healthcare system will not be able to attend to everyone.
- Treatment and prevention – In addition to having a large number of people susceptible to COVID-19, officials also do not know how to treat or prevent COVID-19 since it is such a new virus. In contrast, both antiviral medications and influenza vaccines offer tools to keep large numbers of people from becoming very sick and dying from influenza virus.
Over time, we will learn more about COVID-19, more people will develop immunity, and tools will be developed to defend ourselves against this virus. When that happens, dramatic measures like those we are using in 2020 will be less necessary.
How did this new virus form?
All organisms use genetic material to guide their reproduction. Genetic material can change in two ways during reproduction:
- Replication errors – During replication, sometimes an error is inadvertently introduced. It is called a point mutation. These small errors may have no effect, or they can be significant enough to cause disability or death of the newly replicated progeny.
- Introduction of new genetic material – Sometimes genes from one virus can enter cells and mix with genes from another virus that entered the same cells. The new genetic material can, as described above, have little to no effect, or it can cause a significant change in the virus.
Scientists are still working to understand exactly how COVID-19 developed, but genetic studies have provided some information. More than 95% of the genes are derived from a bat coronavirus. But, a critical part of the genes, related to how the virus attaches to cells, is different. That genetic information is more similar to a coronavirus found in pangolins, a type of anteater. Scientists are still working to figure out how another critical change occurred as well. This change is related to how infectious the virus is and which types of organisms it can infect. Scientists think that either the genes changed in another type of animal, yet to be identified, or this virus was spreading undetected among people without causing illness. Then, while it was reproducing in people, the genetic material changed again enabling the virus to become more infectious.
Related resource:
How Do Viruses Reproduce? (Animation) – This less than 2-minute animation describes how viruses replicate. Versions for elementary, middle or high school/college students are available with voice-overs adapted for each age group.
To see current (2021) content, please visit: The Coronavirus Pandemic – Answering Your Questions.